The Ghosts That Could Change Everything
They appear as little more than ghosts — yet the secrets they tell may soon revolutionise science.
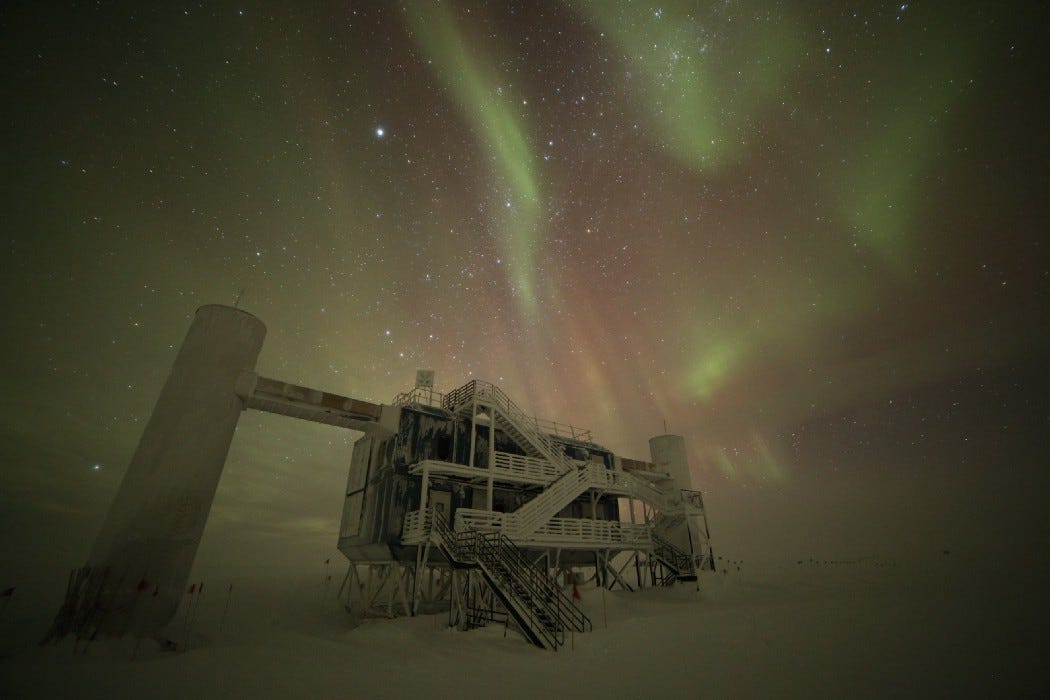
The first sign was astonishingly brief, just thirteen seconds long. It was so brief, in fact, that until much later nobody realised it had happened at all. But something unusual was indeed happening on that February afternoon in 1987, something that hadn’t happened in almost four centuries.
The next sign followed a few hours later, though for some time nobody noticed it either. A photographer in Australia unknowingly captured the event on camera, but wouldn’t realise what he had found until the next day. By the time night fell in Chile, hours later, the sign was bright enough to be seen by the naked eye, if you knew what you were looking for.
Fortunately Ian Shelton did know. But even then, though, it took a moment of disbelief before he reached for the phone. Once he did, however, the news rapidly spread; and with it an army of telescopes and satellites swung into action. They each confirmed with Shelton had seen: for the first time in four hundred years a new star had appeared in the sky.
The ancients regarded such events with awe and superstition. Today, thanks to modern science, we know they mark the death of a giant star; a sudden and powerful bust of energy exploding in a supernova. The event of 1987 was the first opportunity to study one with modern technology; an unprecedented chance to learn more about one of the most extreme events in the cosmos.
The Kamioka Observatory, based in Japan, was alerted by an urgent message shortly after the supernova erupted. Sensational news! Supernova went off 4–7 days ago in Large Magellenic (sic) Cloud… Can you see it? This is what we have been waiting 350 years for!
The scientists at the observatory rushed to check. Can you see it? referred to not to their eyes, but to a groundbreaking experiment. Observations were automated, recorded to magnetic tapes that needed to be retrieved and carefully analysed. With luck their experiment, the Kamiokande, might have spotted the first brief sign of the supernova.
Unlike traditional astronomical observatories, the Kamiokande was not a telescope sitting on top of a high mountain. Instead the experiment was buried deep underground, a tank of water filling an abandoned mine. No starlight could penetrate so deep, but something else, something more ghostly, certainly could.
Dotted around the experimental tank were photon detectors, each calibrated to spot occasional faint flashes of light in the utter darkness of the mine. Normally these flashes were rare. Two or three might be spotted in a week. But if the message was right, then something like fireworks should have been set off as the supernova erupted.
Once they had the tapes, it turned out they had almost missed the event. Engineers had interrupted the experiment for maintenance moments before the explosion. Fortunately, by the time the first turbulent waves from the supernova swept through, Kamiokande was fully operational. A single brief spike showed up in its data, a spike that captured an event nobody had ever witnessed before: one that promised a new era of astronomy.
Over thirteen seconds ten billion neutrinos — a tiny sub-atomic particle — washed over each square centimetre of the Earth. By itself that is not too unusual. Every second trillions upon trillions of neutrinos pass right through the billions of tonnes of rock we call home. A hundred trillion or so are moving through you as you read this. For the most part, though, these particles treat us as shadows.
Neutrinos are small, which is one reason they can fly right through us without stopping. But they also rarely interact with what we would call matter — the protons and neutrons that make up the physical world around us. This interaction is so rare, in fact, that even though countless trillions pass through you every second, only a handful will ever hit you.
Over your lifetime, assuming you live a good few decades, perhaps one trillion trillion neutrinos will pass through you. But they are so ghost-like that of that vast number, less than ten will actually strike an atom in your body.
Spotting neutrinos, then, takes two things: a lot of atoms, and a lot of patience. Experiments like the Kamiokande use tanks of ultra-pure water, essentially gathering lots of atoms in one place, and sensitive detectors to look for the telltale signs of a neutrino passing by. Though collisions are rare, they happen often enough in these tanks to make the experiment worthwhile.
If a neutrino hits an atom in exactly the right way, a tiny explosion results. The unfortunate atom blows up in a shower of particles. As these particles ricochet across the tank they emit Cherenkov radiation — something similar to a sonic boom. The cameras around the tank see this as a faint glow, the sign of a visiting neutrino.
In the Kamiokande experiment, buried deep under Mount Ikeno, only one in every few trillion neutrinos leaves this kind of trace. During the thirteen second supernova blast, Kamiokande spotted just eleven of the hundred thousand septillion neutrinos that hit the Earth. Though it may not sound like much, it was a momentous achievement: one worthy of a Nobel Prize.
The light of a supernova can outshine an entire galaxy: a single dying star burns brighter than a billion other stars combined. But the neutrino flash it generates is somehow even greater, a sign that lights up the universe, if only for a moment.
The flash is created in the dying moments of the star. As the powerful nuclear explosion powering the star comes to an end, its outer layers implode at incredible speed. This implosion is so strong that atoms themselves are crushed — creating a sudden surge of escaping neutrinos.
The resulting shockwave blows up the star, turning an implosion into a supernova. It is brief, seconds in duration, but extraordinarily bright, at least if you can see it. For astronomers this flash is the first sign of a supernova, preceding the light of the explosion by several hours.
So far we have only spotted two, perhaps three, such flashes in the cosmos. But that is partly because we haven’t been looking very hard. At the time of the supernova, in 1987, neutrino detectors were in their infancy. Today’s detectors — including an advanced successor known as the SuperKamiokande and a second powerful observatory at the South Pole — can spot many more neutrinos.
Next time a supernova erupts close to Earth we’ll see thousands of neutrinos. The event will be remarkable, and not just because of the bright new star in the sky. Armed with with the data from these neutrinos, astronomers will be able to probe the hidden secrets of dying stars.
Two key secrets stand out. The first is what, exactly, happens in the first moments of a supernova. We’ve seen the devastating aftermath, and watched the remnants gradually fade away. But we still don’t know how the star blows up. The wave of neutrinos undoubtedly has something to do with it, but just how these fleeting and lightweight particles can hurl billions of tonnes of gas into space is still mysterious.
The extreme conditions at the heart of a dying star will help reveal the second secret. Such places push our theories of physics to their limits — and scientists are hoping that more data about the neutrinos that originate there will uncover new laws of physics.
Unfortunately such supernovae are rare. Before 1987, the last major supernova spotted in our galaxy happened in 1607. Astronomers think they should be more common, perhaps occurring once every fifty years or so. But until one does erupt, physicists will need to be patient.
Luckily, neutrinos may help to explain another long-standing cosmic mystery: the question of what dark matter is. Decades ago astronomers realised that galaxies seemed to spin at the wrong speeds, acting almost as though a large unseen mass was pushing them around faster. Over time the evidence for this invisible, or “dark”, matter has grown stronger, but astronomers have struggled to find out what, exactly, it is.
To fit the observations, there must be a lot of the stuff, far more of it that the familiar objects we can see: the stars, atoms and planets that make up the visible universe. To make it as invisible as it appears, it must be extremely inert. That’s to say, in the language of physics, it must not interact with three of the four fundamental forces of nature.
As a result dark matter emits and absorbs no light, and passes through planets and stars as though they were ghosts. Only via gravity - the weakest of the four forces - can we see any sign of its presence. All this makes dark matter extremely hard to detect on Earth - obviously, since otherwise we would probably have found it before.
What is dark matter made from? Most scientists believe it is some kind of particle, something fleeting and ghostly. Something, indeed, that sounds rather like a neutrino. Yet there is a crucial difference. While neutrinos ignore two of the fundamental forces, they are subject to the other two: the weak nuclear force, and gravity.
But there is another type of neutrino, one still hypothetical, that also ignores the weak force. This, the “sterile neutrino”, would theoretically only feel the force of gravity. They could pass right through the Earth, right through the galaxy, without ever interacting with another particle.
Detecting them is hard, almost impossible. Even the massive HyperKamiokande — the planned successor to the SuperKamiokande — could never hope to find one. The best we can do is look for their absence, a set of missing neutrinos where we might expect some to appear.
And indeed, some signs of these missing neutrinos have been spotted. Two experiments in France found roughly 3% less neutrinos than predicted. Another in China put the missing fraction closer to 6%. But other experiments, including the IceCube Observatory at the South Pole, have found no sign of this missing fraction. For now, at least, its existence is still theoretical; a possibility not ruled out, as far as we know, by the laws of physics.
Could sterile neutrinos account for dark matter? On the face of it they fit the specification: an almost invisible particle that only feels the force of gravity. They have mass — though how much is unknown — and they could quite convincingly fill the universe. If large numbers were created in the Big Bang, which seems plausible, they might still be around today.
So far, however, the evidence is weak. The question of the sterile neutrino is an intriguing one, but one that has so far proved hard to resolve. Experiments coming over the next decade will be able to shed light on their existence, or not, but for now their very presence is little more than speculation.
Even if dark matter turns out to be something else, neutrinos still have a lot to offer astronomers. Neutrino observatories are gradually opening up a new view of the heavens, one that may reveal deep secrets about some of the most extreme places in the Universe. The next supernova, whenever it comes, promises to be the most watched in history.