The Week in Space and Physics #1
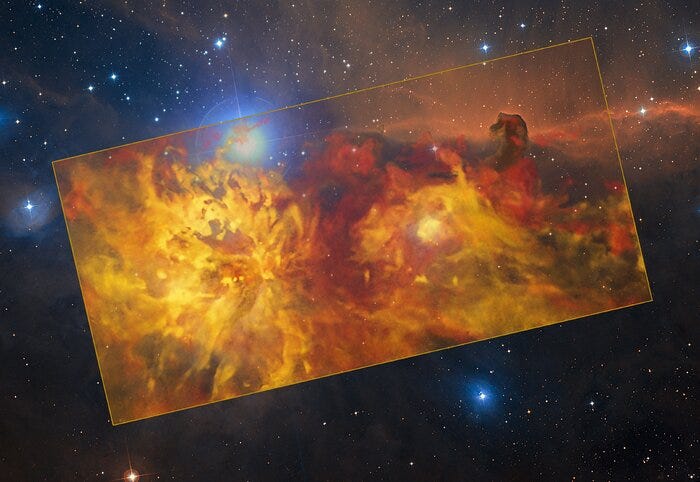
Stars spend most of their lives locked in a delicate balancing act. Two forces, perfectly equal and opposite, constantly battle for control. One – gravity – pushes inwards, seeking to compress the star into an ever smaller volume. The other – a mixture of gas and radiation pressure – pushes outwards, fuelled by powerful nuclear reactions in the stellar core.
These two forces exist in a balance, each supporting and constraining the other. The more gravity pushes; the stronger the nuclear reactions - and hence the outwards pressure - become. Yet remove either force, and the whole system collapses. Without gravity, the star would explode outwards, the reactions would end and the star would cease to shine. Without those reactions, however, gravity would crush the whole thing into a neutron star, or worse: a black hole.
When a star nears the end of its life, this balance begins to break down. As nuclear fuel – mostly hydrogen or helium – runs out, the nuclear reactions become less powerful. Gravity pushes relentlessly inwards, and the star begins to collapse. Sometimes this collapse can trigger a new set of reactions – burning a heavier element like lithium – and stability may be restored for a while.
This cycle comes to an end when a star starts trying to burn iron. Unlike all lighter elements, iron cannot produce enough energy to sustain a nuclear reaction. Pressure, in effect, vanishes – giving gravity a final victory. Triumph rapidly follows: a massive star implodes within hours; matter slamming into its core at incredible speed.
The result is a final, and devastating, nuclear explosion. The core of the star blows apart with tremendous force, shattering the star and igniting a supernova. An intense burst of light follows – a single supernova can outshine an entire galaxy – accompanied by a wave of ghostly neutrinos and a lingering remnant, often in the form of a black hole.
This, at least, is the theory. In practice, capturing a dying star early enough to fully watch a supernova play out is tough. Such events are normally only spotted after the explosion, when the flash of light attracts our attention. That means many of the details of supernova – such as the exact process that triggers them – remain somewhat mysterious.
In a paper published last week, however, a team of astronomers reported watching a star over the last six months of its life. The star - a red supergiant - brightened suddenly earlier this year. That event was probably an explosion of some kind, throwing out clouds of dust. Regardless, it caught the attention of telescopes - and it was imaged several times in the following weeks.
When the star did explode, then, astronomers were ready – and rapidly caught a series of observations. Though the full results will take time to process, it already looks like some new discoveries have come out. Red supergiants, it appears, have many surprises to teach astronomers.
Supernovae and Life: A Strange Connection
Supernova, by long ago seeding the cosmos with heavy elements, played a crucial role in the origin of life. But have they also been meddling in the evolution of that life ever since? At first glance the idea seems faintly ridiculous: supernova, though big, are rare and usually far from Earth. Yet a new study, from Henrik Svensmark at the Danish Technical University, claims to have found a link.
The argument hinges on the effect galactic cosmic rays have on the Earth’s climate. These rays – essentially fast moving protons – are created as shockwaves expand away from supernova. As they hit the Earth their path through the atmosphere triggers the formation of clouds. The more galactic rays that hit the Earth, the thinking goes, the cloudier the Earth will be.
That, estimates show, should impact the climate of our planet. When supernova numbers and galactic ray levels are high the Earth will cool. Winds and ocean currents will get stronger, thus stirring up more nutrients for living creatures to feed on. That should permit more life to thrive on our planet: increasing the amount of biological debris buried deep in oceans or under layers of rock.
By looking at the spread of stars in our galaxy, Svensmark could guess how many supernova have exploded nearby in the last half a billion years. An even longer term estimate – covering three and a half billion years – could be found by tracing brown dwarves; a type of cool and long lasting star.
The results show the rate of supernova may have varied by a factor of three – and that periods with many supernova line up well with ancient ice ages. Svenmark found, too, that levels of organic material buried in long ago oceans seem to match the supernova record. All that suggests supernova do indeed influence our climate, and, perhaps, directed the history of life on Earth.
That trend, however, is a long one. Supernova numbers vary over hundreds of millions of years, meaning their influence can only be seen on such long timescales. Other factors - such as changes in the Earth’s orbit and the motion of continental plates leave a faster varying imprint on our climate.
Antimatter and Gravity
Matter and antimatter should, as far as we can tell, have been created in equal amounts during the Big Bang. Every proton should have a twin anti-proton, every electron an anti-electron. And yet, somewhere along the line, all that antimatter seems to have disappeared.
One possible explanation is a difference in the way gravity interacts with the two types of particle. If, for example, gravity attracted matter but repelled antimatter, that could explain the imbalance we see today. Yet testing this possibility is tricky. Antimatter can only be made in extremely small amounts and gravitational effects are, therefore, small.
In an effort to address this, an experiment at CERN, the European particle collider, recently monitored oscillations of protons and anti-protons over the course of a year and a half. Experiments of this can show the mass to charge ratio of the two particles, a ratio that should be the same in both matter and antimatter.
The results indicate that the ratio is indeed identical, at least to within a billionth of a percent or so. But, since the gravitational force on the Earth shifts over a year, physicists could also look for the effect of this force on the particles. Again, no difference was found between the two particles - at least to within 3%.
James Webb Status
Despite more than three hundred possible failure points, the deployment of the James Webb Space Telescope seems to have gone to plan. Over the past week operators unfurled and tightened the sunshield. That allowed them to start cooling instruments onboard the telescope to just forty degrees above absolute zero.
The final, and most challenging, step took place over the weekend: deploying and latching segments of the telescope’s giant mirror. This, too, seems to have gone exactly as planned, with the positions of the eighteen segments now carefully and precisely arranged.
With these crucial steps behind them, mission operators will now guide the telescope towards its final orbit. One more course correction manoeuvre will take place later this month. After that astronomers will almost be ready to start capturing the telescope's first images.