The Week in Space and Physics: A Neutrino to Remember
On ultra-high energy neutrinos, black hole bullets, nuclear fusion and an Einstein Ring.
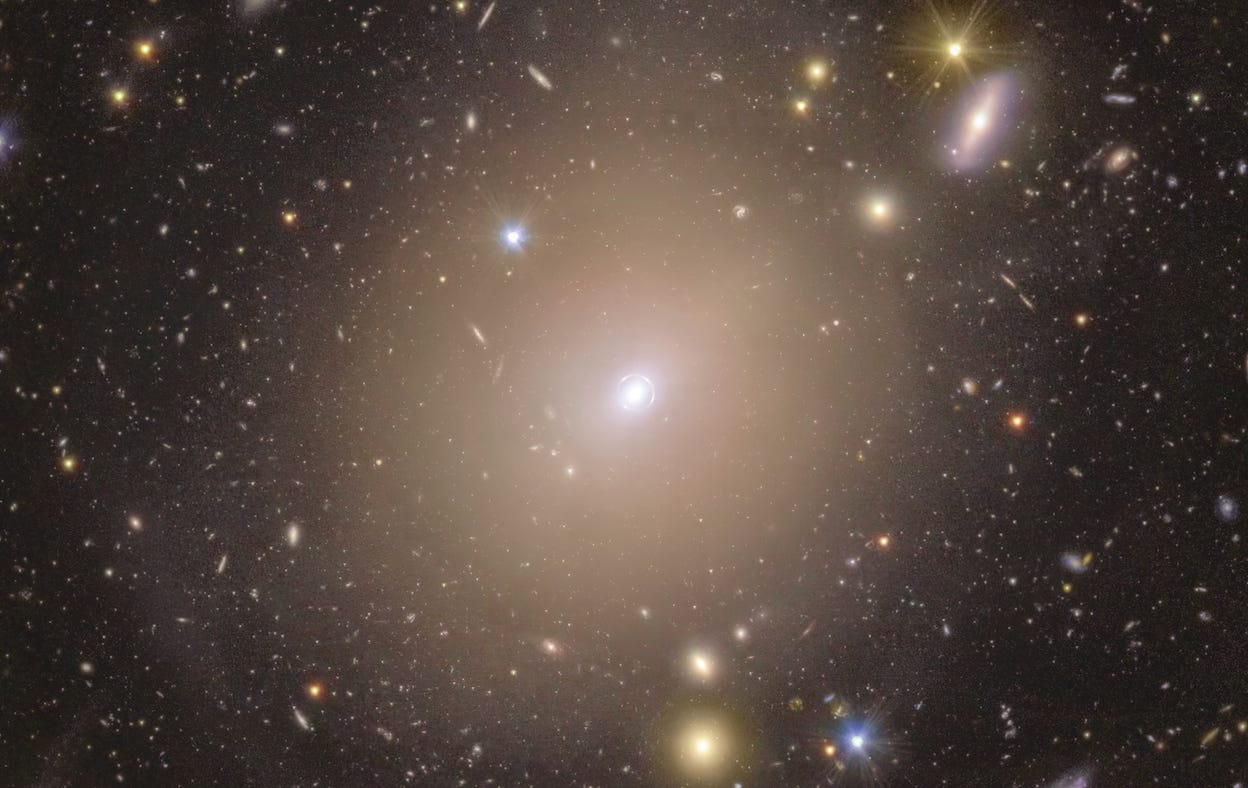
In February of 1987 we saw a supernova erupt in a nearby galaxy. Briefly it was visible to the naked eye, the first such dying star to be so in four centuries. Yet before it was visible in light, it was also visible in neutrinos - and it was the first supernova ever to be seen in these still somewhat mysterious particles.
Not that it was all that easy to see. The neutrino wave came around two hours before the first photons from the supernova washed over our planet. It lasted no more than a few seconds, yet within that time trillions upon trillions of neutrinos must have passed through the Earth. Our detectors saw just twenty-five of them.
Neutrinos, clearly, are elusive beings. They rarely interact with the atoms that make up our world, and can thus pass through the entire planet as though it were little more than a shadow. Around a hundred trillion are probably passing through your body right now, yet over your entire lifespan, just one or two of them will actually hit it.
So it was big news when, on February 13th, a high energy neutrino was spotted moving through the Mediterranean Sea. The particle was seen by a partially completed detector designed to monitor a cubic kilometer of sea water for incoming neutrinos. Whenever such a particle collides with an atom in that volume of water, it produces a shower of other particles and a flash known as Cherenkov radiation.
The detector itself consists of hundreds of sensors strung through the sea. Each watches for the faint signs of those Cherenkov flashes, and thus for the telltale signature of a passing neutrino. Whenever it spots a flash that matches, it is then able to reconstruct the details of the incoming particle.
This all gives the observatory a way to monitor neutrinos coming from astrophysical sources. Yet the event of February 13th was big news even for a detector designed to look for such things. It was the highest energy neutrino ever detected, carrying thirty times more energy than the previous record holder and a thousand times more than any neutrino we are capable of creating on Earth.
The obvious question is where it came from. Such a high energy particle may have been created by a cataclysmic event of some kind, like a gamma ray burst or around an active black hole. Yet observations of its likely direction of travel show no obvious origin. For now, sadly, its source is likely to remain a mystery.
Neither was it clear if this was a lucky one off, or a sign of future discoveries to come. The Mediterranean detector is big, but it is not the only in the world capable of spotting particles like this. Another detector, located at the South Pole, has spent ten years monitoring neutrinos, and never seen any as energetic as this. Indeed, researchers estimate - based on our current understanding of the cosmos - that such a powerful neutrino should only be detected once every seventy years or so.
Black Holes and Human Bodies
When we think of black holes, we usually think of mammoth objects like those depicted in the movie Interstellar, or of those that lurk in the hearts of galaxies. And indeed, we do know there are a lot of black holes like this, and that they play an important role in shaping the cosmos.
But nothing says a black hole has to be big. According to some theories of physics, the Big Bang could have produced a flood of tiny black holes. These would have stuck around - and if the theory is right, the modern cosmos should be full of tiny black holes, each no bigger than an atom of hydrogen and weighing no more than a small asteroid.
That, in many ways, is an attractive idea. Such small black holes would be invisible, hard to detect by other means, and potentially account for much of the universe’s mass - all of which makes them sound rather like dark matter.
But, of course, there are some limits on the possibility. Black holes evaporate over time through a process called Hawking Radiation, and so the smallest of them should already have vanished from the universe. That, studies estimate, rules out anything with less mass than a small asteroid.
But anything much larger than this would begin to create other effects. If there are hordes of black holes the size of small planets, for example, we would observe their disruptive impact on stars, spot the gravitational waves they would make, and see an effect known as microlensing. But since we don’t see these effects, we can assume such black holes don’t exist in large numbers.
That still leaves a window of possibility. Nothing yet rules out large numbers of black holes with roughly the mass of a small asteroid squeezed into the volume of a hydrogen atom. But as one recent paper discussed, if these black holes are really present in large numbers, we might expect to see one colliding with the Earth from time to time.
What might such a thing look like? Some studies have considered the kinds of seismic waves that might be generated. But this paper takes things a step further, and asks what might happen if such a black hole passed through a human body.
The gravitational effects of such a black hole, it concluded, would be minimal. More troubling would be the shockwave of its passage: a small black hole, even one the size of a hydrogen atom, would act something like a bullet passing through a person. You’d probably notice, in other words, if you were struck by one.
That might seem to narrow the possibilities for primordial black holes. Yet their calculations also suggest that black holes collisions with people should be extremely rare, even if they do account for all the dark matter in the universe. You’d have to be very unlucky, in other words, to die from injuries caused by a black hole.
Plasma in the EAST and the WEST
In January, a fusion reactor in China, EAST, became the first ever to maintain a plasma for over a thousand seconds. The achievement, which required controlling plasma heated to close to seventy million degrees Celsius, marked an impressive milestone on the road to controlled nuclear fusion.
Yet the record did not last long. On February 12, a reactor in France, WEST, maintained plasma for even longer, keeping it under control for more than twenty-two minutes. That was about twenty-five percent longer than the Chinese tokamak had managed.
Controlling plasma is one of the hardest challenges of nuclear fusion. It must be maintained at an extraordinary temperature, in some cases reaching a heat greater than that found in the core of the Sun. No material can contain such temperatures, and so fusion reactors must use complex magnetic fields to control and maintain plasma in a state that allows fusion to occur.
Both experiments were conducted as part of studies to aid the development of ITER, a large scale fusion reactor now under development in the south of France. The two successes are a good sign, according to Alberto Loarte, the head of ITER’s Science Division, showing that we have a mature understanding of the technology needed for long tokamak operation.
ITER, when it opens, should be capable of generating up to ten times the amount of energy put in. That could make it the first place on Earth to successfully control fusion in a way practical for energy generation. Yet getting there will take a lot of time and money: ITER is not expected to open before 2033.
A Spectacular Einstein Ring
Europe’s Euclid telescope discovered an almost perfect Einstein Ring in a nearby galaxy. The phenomenon, predicted by the theory of general relativity, has been seen in many places throughout the cosmos. Yet examples as close and as symmetrical as this one are rare.
Einstein Rings form thanks to the distortions in space and time created by large masses like galaxies. This can sometimes result in a lensing effect, where light rays coming from objects behind the mass are magnified and curved. In some cases this distorts a galaxy or star so that it appears as an arc of light instead of as a point. In an Einstein Ring that is taken to an extreme, so that the arc bends into a full circle.
The recent discovery was made in a galaxy already known and catalogued by science. Yet until now it was believed to be a rather boring galaxy - the discovery, indeed, came only by chance as astronomers studied Euclid’s calibration data. More Einstein Rings will certainly be discovered by the telescope in the years to come. But none is likely to be as perfect or as close as this one.
I dunno why, but the poet in me loves reading about neutrinos. Thank you for writing all this down.
A fate arguably worse than death would be to die by black hole collisions, but no one being able to identify the cause. It would be a missed opportunity for an incredible obituary.