The Week in Space and Physics: A Trio of Cosmic Mysteries
On the mysteries of dark matter, the expanding universe, and the missing antimatter.
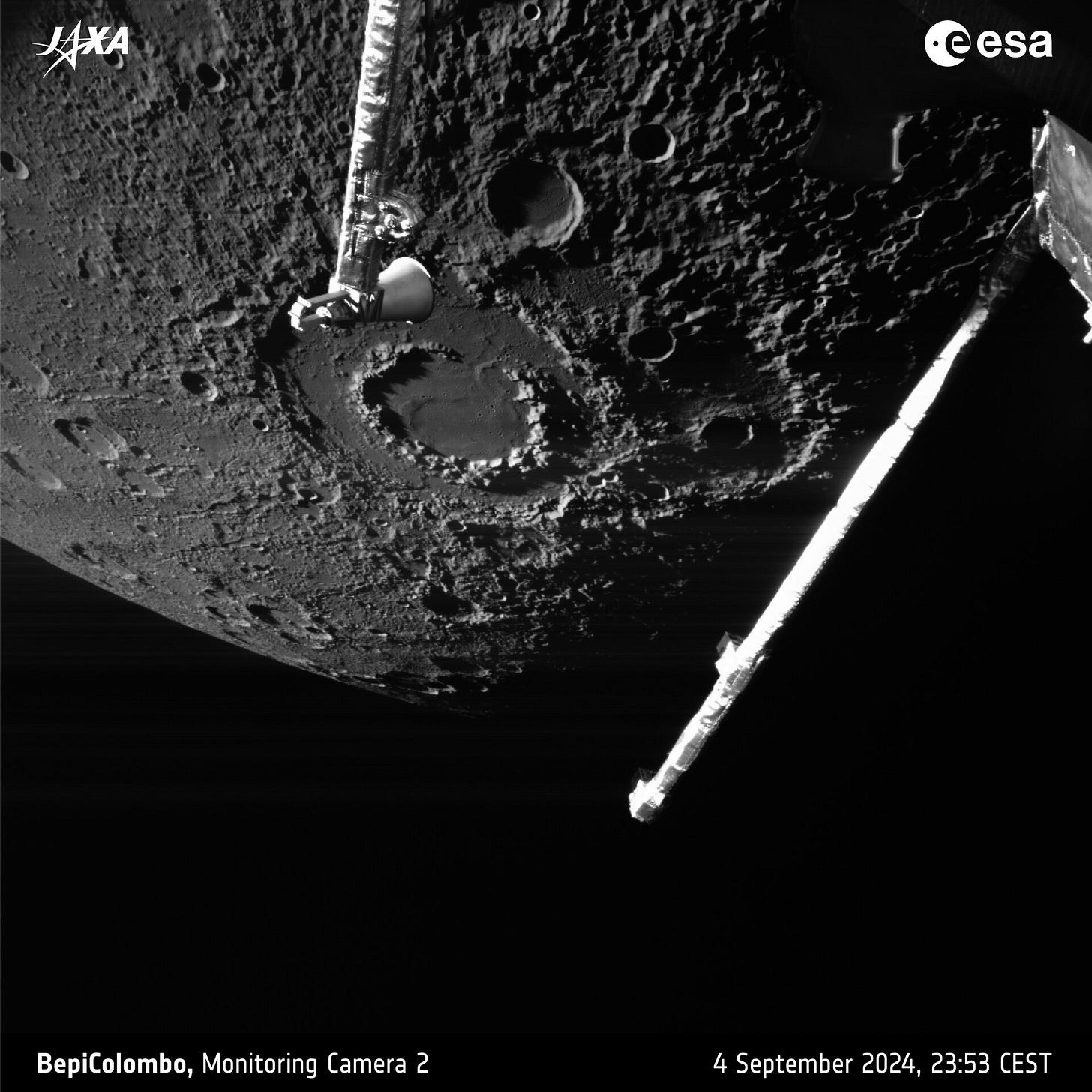
Ask an astronomer, and they may well tell you the question is settled. The effects of dark matter are so clearly visible - in everything from the spin of galaxies to the evolution of the cosmos - that we cannot possibly deny its existence. What it actually is seems irrelevant - and anyway, that question should be directed to the particle physicist, not the astronomer.
Perhaps. Indeed, the answer is not really wrong. We can see the effects of dark matter, and by including a healthy dose of it in our models we can make accurate predictions about the cosmos. But it is also not very convincing. The fact is, and however we cut it, there must be an awful lot of dark matter out there. Our failure to actually find any of it here on Earth is thus rather troubling.
Turn anyway to the particle physicist, and they’ll have plenty of speculative ideas, if little in the way of hard evidence. Dark matter might, they say, be made of hypothetical axions, or of sterile neutrinos, or of dark photons, or any of a dozen other possibilities, none of which have ever been seen or detected.
One of the more likely possibilities is a class of particles known as WIMPs. These, Weakly Interacting Massive Particles, only interact with matter through gravity and the weak nuclear force (“weakly interacting”), somewhat akin to neutrinos. Unlike neutrinos, however, they would be heavy (hence “massive”) and able to interact with each other. They could thus form the kind of cold, slow moving particle needed to explain astronomical observations of dark matter.
If WIMPs exist we should be able to detect them. In an attempt at doing this, researchers have built an underground observatory in an old South Dakota mine. There they have filled a large tank with liquid xenon and placed detectors to spot signs of WIMPs colliding with atomic nuclei. In a sense this is similar to a neutrino detector. Indeed, both look for signs of rare weak nuclear interactions, though WIMP events are expected to be far rarer than neutrino ones.
In a pair of recent presentations, researchers from the observatory showed the results of more than two hundred days of study. No WIMPs were found, although they did see signs of some other rare nuclear interactions.
That’s not all bad news. The results do at least let researchers place new limits on the properties of WIMPs, and so to rule out some possibilities. That will influence the design of future experiments - and, indeed, the observatory in South Dakota will keep on running for a few more years yet.
But the result also does nothing to confirm dark matter actually exists. And it does not rule out a nightmare scenario: that dark matter, if it is there, ignores the weak force too, and acts only via the force of gravity. Should that be the case, detecting any here on Earth would become all but impossible.
An Atom of Antihydrogen
We are all familiar with matter - at least, of the non-dark kind. Everything we can touch, smell and see is made of matter - of atoms, of electrons, of quarks, protons and neutrons. And yet a century ago, as he worked to combine Einstein’s ideas with those of quantum theory, the physicist Paul Dirac realised this is only half the story.
Every particle of matter, he deduced, should have a corresponding particle of antimatter. For every electron there is a positron, for every proton an antiproton. At the time, this was a purely theoretical discovery; a rather strange consequence of pursuing mathematical beauty over pure empirical research.
Four years later, however, Anderson discovered the positron, and Dirac was proven correct. It was a triumph of theoretical physics, but one that also raised troubling questions about the fundamental laws of nature. Those laws, as worked out by Dirac and others, treat matter and antimatter in the same way. Both should thus have been created in equal amounts in the Big Bang, and both should have evolved in the same way ever since.
Yet ours is a matter universe. Observations have shown little sign of antimatter: we have seen no stars burning anti-atoms, nor any galaxies made of anti-stars. Somewhere along the way, then, the universe seems to have lost its antimatter. That implies a subtle difference must exist in the laws of physics, some asymmetry in matter and antimatter that allowed one of them to dominate.
So far we have not found this asymmetry. But if it exists, then particle colliders may be one place to find it. When physicists smash particles together, they create bursts of new ones, including those of antimatter. The quantities are small, and the particles are short-lived, but they at least offer a chance to study the stuff in a lab.
At the end of August, in a paper published in Nature, researchers at the STAR Collaboration of the Brookhaven National Laboratory reported creating the heaviest yet antiparticle. It is, they say, a heavy nucleus of antihydrogen - that’s to say, an antiproton bound to three antineutrons. Yet in this case one of the antineutrons was also “heavy”, with one of its usual down quarks replaced by a heavier strange quark.
Just sixteen of these nuclei were detected, they said, but it is the first time that such heavy antimatter particles have ever been seen. The researchers were also able to study how these particles, as well as some smaller ones, broke apart and decayed into others.
Nothing unexpected was seen. The decays followed exactly the pattern predicted by the laws of physics - and so no sign of the hoped for asymmetry emerged. The mystery of the vanishing antimatter, it seems, must linger on a little longer.
The Hubble Report
Hubble gets the credit for discovering the expansion of the universe, but in truth there were others - Friedmann, Lundmark and Lemaître among them - who realised that fact before him. Nevertheless it is of Hubble’s law we usually speak, and of the Hubble constant too.
That law, simply put, says the further something is from Earth, the faster it is moving away from us. This is a linear relationship, so that something twice as far moves away twice as fast. The rate at which this speed increases is known as the Hubble constant, and measuring it accurately has become something of an obsession in astronomy.
In principle this should be easy enough. All you need to do is measure the distance to faraway objects, measure how fast they are moving away from us, and then plot the resulting data on a graph. The slope of the line relating distance and speed gives you the value of this constant.
Trouble is, it is not always easy to measure the distance to things, especially those that are a billion light years or more away. Astronomers have found various tricks, based on the brightnesses of supernovae and of certain kinds of stars. Yet the accuracy of these methods is limited to the data we can collect from telescopes.
In 2001, based on measurements with the Hubble Space Telescope, astronomers arrived at a value of 72 km/s/Mpc for the constant (that is, objects separated in distance by one megaparsec - roughly three million light years - move apart at 72 km/s). In 2011 that number was refined slightly to 73 km/s/Mpc.
In 2013, however, researchers used another telescope - Planck - to look at radiation coming from soon after the Big Bang. In doing so they were able to derive many fundamental parameters of the cosmos, including the Hubble constant. Their result, however, was much lower than expected, coming in at only 67.4km/s/Mpc.
Although Hubble surely has enough things already named after him, this difference has become known as the Hubble tension. Possibly it points to something fundamental about the universe - some unexpected variation in how it has expanded over time. But, perhaps more likely, it points to some error in our measurements and models.
New data from the James Webb telescope seems to strengthen the case for the latter. With its gaze, astronomers have been able to pick out distant stars more clearly, and so measure their distances more accurately. Based on that new data, some researchers now think the Hubble constant is lower than that found by the Hubble telescope.
Whether this is enough to resolve the Hubble tension remains to be seen. But it does seem plausible astronomers will settle the question in the coming years, and may finally have the data and observations needed to pin down exactly how fast the universe is expanding.
Starliner Returns At Last
After three months docked at the International Space Station, Boeing’s Starliner capsule touched down in New Mexico last Friday. The landing marked the end of an extended test flight for the capsule, one which sadly raised new questions about its readiness to carry astronauts safely.
Under the original plan, Starliner would have carried a pair of astronauts to the station and back, and, after it returned, have been certified as ready to begin routine flights. Instead issues with the capsule’s thrusters appeared as it neared the station. The initial two week stay there was extended, and then extended again, as engineers sought to prove it safe enough to bring the astronauts back.
For NASA, however, the risks were too high. Instead of bringing its crew home, Starliner returned empty last week. And though the landing went smoothly, some issues with its thrusters were indeed seen during the return. Barring a cancellation of the project - which is by no means out of the question - NASA and Boeing will now work to fix these issues, and then move towards another flight sometime at the end of next year.
Have WISPs been observed anywhere prior to their attribution to dark matter or are these theoretical particles that are thought to describe dark matter?
Oh, , here goes with the armchair expert and his pronunciamentos!
1. Balance- Asymmetry in the Big Bang, a miniscule initial difference that magnified over time
A very natural consequence of the pursuit of mathematical or theoretical beauty being misleading. Nothing is perfectly symmetric over time!
2. Dark matter- forget WIMPs, think neutrinos! Lots and lots of neutrinos!
3. Expansion- Something's wrong with the standard candle! In fact it would be quite surprising if symmetric expansion was proceeding uniformly! More likely not!
Okay that's that. All is solved satisfactorily. Tea time! Oi! My crumpet is not round!